Introduction
Materials and Methods
Plant Growth and the Plant Factory
(NH4)2SO4 Treatment
(NH4)2SO4 and LED Treatment
Sample Extraction, Purification, and Instrumental Analysis for Assay of GSL Content
Statistical Analysis
Results and Discussion
Growth Characteristics of Chinese Kale using Different (NH4)2SO4 Concentrations under a Single LED (B0:R1:W12) Condition
Growth Characteristics of Chinese Kale Owing to Different Combinations of (NH4)2SO4 Concentrations and LEDs
Content and Types of Glucosinolate in Chinese Kale Owing to the Combination of (NH4)2SO4 and LED
Introduction
Recently, consumers have become interested in different vegetables that possess health-promoting effects and immune activity. Among vegetables, those that are cruciferous, such as kale (Brassica oleracea var. acephala DC.), Chinese kale (B. oleracea var. alboglabra Bailey), red cabbage (B. oleracea var. capitata f. rubra L.), brussels sprouts (B. oleracea var. germmifera Zenker), kohlrabi (B. oleracea var. gongylodes L.), and broccoli (B. oleracea var. italic Plen), are rich in glucosinolates (GSLs) (Kushad et al., 1999; QIA, 2019). Plants of the Brassicaceae family that contain GSLs (i.e., more than 100 secondary metabolites in plants containing sulfur and nitrogen), are agriculturally important crops (Fenwick and Heaney, 1983; Ivana et al., 2008). Among them, Chinese kale is a representative of B. oleracea that contains a large amount of GSLs (Xin et al., 2015).
GSLs can be divided into three groups depending on their amino acid precursors and structure: the first group includes aliphatic GSLs derived from methionine, isoleucine, leucine, or valine; the second group is comprised of aromatic GSLs derived from phenylalanine or tyrosine; finally, the third group contains indole GSLs derived from tryptophan (Wittstock and Halkier, 2002). Among the different GSLs, sinigrin (SIN, allyl-glucosinolate, or 2-propenyl-glucosinolate) was reported to have health effects that are beneficial to human nutrition (Fahey et al., 2001). GSLs exert anti-cancer effects by inducing the activity of phase II detoxification enzymes. The hydrolysis of SIN is catalyzed by myrosinase enzymes (Teklehaymanot et al., 2019).
SIN is a natural aliphatic GSL present in plants of the Brassicaceae family, such as broccoli and brussels sprouts, and mustard seeds. Since ancient times, studies conducted on the pharmacological activities of SIN have revealed its anti-cancer, antibacterial, antifungal, antioxidant, anti-inflammatory activities, wound healing properties, and biofumigation effects (Mazumder et al., 2016). However, in leafy vegetables, its concentration widely varies and is dependent on genotype, plantation, and food recipes (Possenti et al., 2017). GSL content is also dependent on environmental factors, plant parts, the physiological stages of plant growth, and the level of insect damage (Velasco et al., 2007; Pedras et al., 2011). Jóska et al. (2009), suggesting that increasing the concentration of SIN in plant nutrition would be desirable by controlling fertilizers, such as nitrogen and sulfur.
By examining the relationship between sulfur-containing compounds and plants, during the winter season, the temperature in greenhouses is maintained with a heating system that uses sulfur-containing fuel that emits sulfur dioxide. High concentrations of sulfur can damage vegetables in greenhouses and potentially harm farmers if its concentration in the air is poorly controlled (Kim et al., 2011). Nevertheless, when present at low levels, sulfur has a positive effect as it is a major nutrient for plant growth (Darrall, 1989; Kim et al., 2011; Ashraf and Harris, 2013; Lee et al., 2017). Sulfur is absorbed as sulfate through the roots and leaves and is used to synthesize indispensable compounds such as cysteine, methionine, glutathione, sulfur-containing acids, and GSLs, which are the main functional compounds discussed in the present study. Sulfur also plays an important role in growth, and the physiological and biochemical processes in plants. Nitrogen is an important factor that influences GSL synthesis and content. In Brassica studies, GSL was found to increase in the presence of a sufficient sulfur concentration and high nitrogen supply (Zhao et al., 1994; Ahmad et al., 2007; La et al., 2009).
Plant factories have presently captured the spotlight as consumers are demanding safe food and functional vegetables to achieve good health, and farmers need a stable supply. A fully controlled plant factory that can be certified as an eco-friendly agricultural production facility is managed by using a hydroponic cultivation system and an artificial light source. In a plant factory, high pressure sodium light sources, which generate an abundance of heat due to space constraints, are difficult to use. However, fluorescent and light-emitting diodes (LEDs) are frequently employed as light sources to improve the light energy utilization efficiency because they can provide the illumination required by plants (Toyoki, 2007). LEDs have also functioned as suitable light sources for plant growth chambers based on their small weight and volume (Bula et al., 1991; Bartra et al., 1992).
Different GSL contents in kale shoots were observed with different colors of single LEDs.GSL content gradually decreased in Chinese kale seedlings under red light after transplanting (Qian et al., 2016). Changes in the phytochemicals of broccoli and GSL biosynthesis of Arabidopsis thaliana according to the photoperiod were also reported (Huseby et al., 2013; Steindal et al., 2013). SIN, an aliphatic GSL, significantly responded to two wavelengths in the kale shoot tissues. In particular, the 640-nm and 730-nm wavelengths showed high SIN content but other wavelength treatments did not affect the accumulation of SIN. Moreover, the wavelength was found to have no effect on the accumulation of any GSL, except SIN (Lefsrud et al., 2008). Therefore, we assume that the change of sulfur and wavelength will be the improvement of GSLs in the plant factory system to enhance the functional compounds. The aim of the present work was to investigate the growth characteristics and GSL levels of Chinese kale within a plant factory using four concentrations of ammonium sulfate [(NH4)2SO4], added as a nutrient additive to the nutrient solutions, and four different combinations of LEDs.
Materials and Methods
Plant Growth and the Plant Factory
Chinese kale (Brassica oleracea var. alboglabra Bailey cv. Jellujon) was purchased from Asia Seed Korea (Seoul, Korea). All experiments were carried out using the nutrient film technique (NFT) and hydroponic equipment in a plant factory (Insungtec Co., Yongin, Korea). The environmental conditions in the plant factory were controlled at 22.5 ± 2.5°C and 80 ± 5% relative humidity. The light cycle was 13/11 h day/night. Chinese kale plants were grown for 2 weeks under white light after sowing in a 162-cell plug tray. Plants were transplanted into the NFT beds at bays in the plant factory at 2 weeks after sowing, and then harvested at 4 weeks after transplanting to investigate their growth characteristics and analyze the GSLs.
(NH4)2SO4 Treatment
The nutrient solutions used in this experiment are presented in Table 1. (NH4)2SO4 was adjusted to contain four levels of NH4 ‑ N and SO4 ‑ S [NH4 ‑ N + SO4 ‑ S = 1.0 + 0.8 mM (S1), 1.1 + 0.9 mM (S2), 1.2 + 1.0 mM (S3), 1.4 + 1.1 mM (S4)], respectively (Table 1). The S1 ‑ S4 treatments were set to EC 2.0 dS·m-1. The purpose of the first experiment was to select an appropriate range of (NH4)2SO4 concentrations to enhance the GSL content of Chinese kale, without harming the growth characteristics.
Table 1.
Comparison of nutrients among the nutrient solutions (S1-S4) containing four different (NH4)2SO4 concentrations, PBG, and Yamazaki (unit: mM)The first experiment was conducted using one type of LED (B0:R1:W12, blue:red:white ratio = 0:1:12) and four different concentrations of (NH4)2SO4 (S1 ‑ S4) at four bays, respectively. All nutrient solutions were supplied in a circulating manner. At 2 weeks post-sowing, 21 seedlings per block were planted in 2 blocks to achieve a total of 42 seedlings per concentration treatment. Growth characteristics, including plant width, plant height, the number of leaves, leaf width, leaf length, leaf thickness, chlorophyll content using single photon avalanche diode (SPAD), and leaf fresh weight (LFW) were investigated, and the leaf traits were measured in three normal leaves per individual.
(NH4)2SO4 and LED Treatment
The second experiment was conducted to determine the most suitable combination [i.e., the four (NH4)2SO4 concentrations (S1 ‑ S4) and the four LED conditions] for enhancing the GSL content of Chinese kale. The LED (DMLED Co., Ltd., Ansan, Korea) consisted of a combination of three LED colors (B: blue; R: red; W: white) which were combined to create the following 4 LED conditions: B0:R1:W9 (128 µmol·m-2·s-1, 20W), B2:R3:W5 (119 µmol·m-2·s-1, 20W), B0:R5:W5 (128 µmol·m-2·s-1, 20W), and B0:R1:W12 (136 µmol·m-2·s-1, 24W). The nutrient solutions containing four different (NH4)2SO4 concentrations as same as described in the first experiment were supplied in a circular manner to each of the four bays, and four LED treatments as mentioned above paragraph were installed with two blocks per bay. At 2 weeks after sowing, 21 seedlings per block were planted in two blocks to achieve a total of 42 seedlings per combination treatment using four (NH4)2SO4 concentrations and four LEDs.
The growth data were the same as those described in the first experiment. To analyze the content of GSL per combination treatment, the leaves of Chinese kale at 4 weeks after transplanting were lyophilized in a freeze-dryer (Ilshin Bio Base Co., Ltd., Dongducheon, Korea) for 48 h.
Sample Extraction, Purification, and Instrumental Analysis for Assay of GSL Content
Briefly, 5 mL of hot 70% MeOH solution was added to 200 mg of the lyophilized sample, and extracted via heating at 80°C for 10 min. An additional 30 min of sonication was performed as part of the extraction. The strong anion exchange (SAX) cartridge (500 mg, 6 mL) was activated with 2 mL of 70% MeOH. Thereafter, 2 mL of the extract was added. Each cartridge was washed with 1 mL of distilled water and 2mL of acetate buffer (pH 4), followed by the addition of 1 mL of sulfatase solution (0.2 mg-1·mL-1).The reaction was carried out for ≥16 h to remove the sulfate group. After completion of the reaction, the desulfonated GSLs were eluted with 1.5 mL of distilled water into HPLC vials for analysis.
Desulfoglucosinolates were analyzed by HPLC with detection at 229 nm (Shimadzu, Japan). Table 2 shows the instrumental conditions used for the analysis. Identification of each desulfoglusosinolate was performed using HPLC- MS-MS. The molecular ion and fragment ion were confirmed in positive mode with a voltage of ‑15V CE. To identify the glucosinolate compound, a neutral loss spectrum of the glucose fractions was employed. The HPLC MS-MS conditions are presented in Table 3.
Table 2.
Analysis conditions used to detect glucosinolates in Chinese kale for sample extraction and purificationTable 3.
HPLC-MS/MS conditions for the glucosinolate analysis of leaves from Chinese kaleStatistical Analysis
First experiment and analyses were conducted in a completely randomized design with 20 individual plants for each treatment. Second experiment was the same as the method above for each treatment, but 15 individual plants were used for statistical analysis. Data were assessed using analysis of variance (ANOVA) with the SAS 9.2 software (SAS Institute Inc., Cary, NC, USA) and means were compared by Duncan's multiple range test, with a significance level of 5%. The graphical results were generated with SigmaPlot 10.0 (USA).
Results and Discussion
Growth Characteristics of Chinese Kale using Different (NH4)2SO4 Concentrations under a Single LED (B0:R1:W12) Condition
After treating Chinese kale with (NH4)2SO4 for 4 weeks after transplanting, we identified significant changes in plant width, leaf length, leaf width, leaf thickness, and LFW; however, plant height, chlorophyll content, and the number of leaves were not significantly different (Table 4). Treatment with S3 caused the greatest increase in plant width, leaf length, leaf width, and leaf thickness among all (NH4)2SO4 concentrations tested. S3 resulted in an average LFW of 74.1 g, thereby resulting in approximately 10 ‑ 30% better growth than the other treatments; it was followed by S2, S4, and S1. The LFW of Chinese kale was estimated to be directly affected by leaf size, and not the plant height and number of leaves.
Table 4.
Growth characteristics of Brassica oleracea var. alboglabra Bailey cv. Jellujon influenced by (NH4)2SO4 at the harvest stagezyMean separation within columns by Duncan's multiple range test at p = 0.05.
ns,***Non-significance or significance at p ≤ 0.05 or 0.001, respectively.
At high concentrations of sulfur, there is either a change in leaf size and shape, or a reduction in plant growth (Royer et al., 2005; Bacon et al., 2013). Maintaining sulfur concentration and increasing nitrogen supply was found to delay the growth of Indian mustard (Jóska et al., 2009). In addition, higher concentrations of sulfur can cause damage in younger and older leaves than in mature leaves (Iqbal et al., 2014). Even with a sufficient supply of nitrogen, plant height was found to decrease because of a shortage in sulfur (Jóska et al., 2009). The “Law of the Optimum” states that the closer the other element is to the optimum, the more effective the absorption of the other element (Wollny, 1887; Liebscher, 1895). In this study, the purpose of (NH4)2SO4 treatment was to increase the GSL content and to determine how it affects the growth characteristics, as a strong interaction can be expected between nitrogen and sulfur supply for GSL content (Jóska et al., 2009). In a preliminary experiment, Chinese kale either died, or its growth was retarded when it was treated with an (NH4)2SO4 concentration higher than S4. Therefore, the highest level of (NH4)2SO4 was set to S4 in this experiment (Table 4). Altogether, the concentration range of (NH4)2SO4 employed in this experiment provided a balanced supply of nitrogen and sulfur and did not negatively affect the growth characteristics.
Growth Characteristics of Chinese Kale Owing to Different Combinations of (NH4)2SO4 Concentrations and LEDs
By employing 16 combinations created with four (NH4)2SO4 concentrations and four LED conditions, the growth characteristics of Chinese kale at 4 weeks after transplanting were found to significantly increase the plant width, plant height, leaf length, leaf thickness, chlorophyll content, and the number of leaves, except for leaf width (Table 5). Regardless of the (NH4)2SO4 concentrations, B0:R5:W5 caused the best increase in plant width, plant height, leaf length, leaf width, leaf thickness, and the number of leaves, but not in chlorophyll content, among the four LED conditions. Although B2:R3:W5 caused the highest value for chlorophyll content, all other growth characteristics were lower than those obtained with the other LED treatments.
Table 5.
Growth characteristics of Brassica oleracea var. alboglabra Bailey cv. Jellujon influenced by the combination of (NH4)2SO4 and LED at the harvest stagezyMean separation within columns by Duncan's multiple range test at p = 0.05.
ns*,***Non-significance or significance at p ≤ 0.05, 0.01, or 0.001, respectively.
The LFWs of Chinese kale exhibited no significant changes with LED treatment, but displayed highly significant differences with (NH4)2SO4 concentrations (Table 5). Moreover, S2 and S3 treatments showed the greatest LFWs under B0:R5:W5. As shown in Fig. 1, other (NH4)2SO4 concentrations ranging from S2 to S4 caused a greater increase in LFW than S1. As a result, the growth of Chinese kale owing to (NH4)2SO4 concentrations was similar to that obtained in the first experiment. Thus, the growth responses caused by the light source were examined.
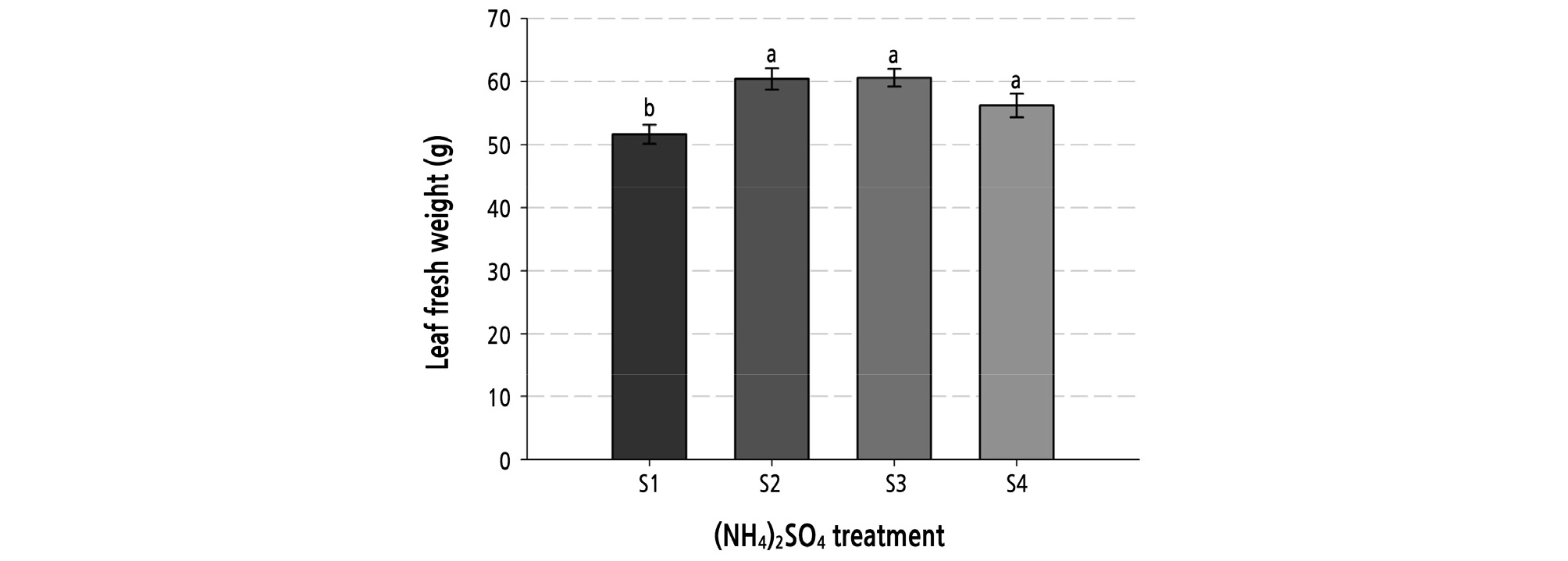
Fig. 1.
The leaf fresh weight of Brassica oleracea var. alboglabra Bailey cv. Jellujon influenced by the four different (NH4)2SO4 treatments in the hydroponic culture. Different letters within the same cultivar indicate significant differences at p = 0.05 by Duncan’s multiple range test. Values shown refer to those from Table 1.
Xin et al. (2015) reported that a combination of red and blue LEDs is optimal for the growth of Chinese kale (cv. Lvbao). The highest shoot biomass was achieved with more red LED (B1:R8:W0), while the lowest was achieved with more blue LED (B3:R6:W0). However, Lee et al. (2016) reported that the LFW of kale (B. olearacea L. var. acephala) exposed to B1:R0:W1 combined with a fluorescent lamp (FL) at 6 weeks after transplanting was greater than that of kale exposed to B1:R0:W1 and B1:R2:W3. They suggested that the growth of kale was maximized by enhancing the continuous wavelength of FL. In the LED comparison of lettuce (Lactuca sativa L. cv. Fidel) at 5 weeks after transplanting, B0:R5:W5 resulted in the highest shoot FW, with a statistically significant difference found relative to B0:R1:W9, B2:R3:W5, and B0:R1:W12 (Song et al., 2019). Based on our results, red wavelengths, and not white or blue wavelengths, were considered to have a positive effect on the major growth factors that determine the productivity of Chinese kale, regardless of light intensity. Overall, S2 and S3 treatments were found to enable the best growth under B0:R5:W5 than other combinations of (NH4)2SO4 concentrations and LEDs.
Content and Types of Glucosinolate in Chinese Kale Owing to the Combination of (NH4)2SO4 and LED
Six major GSLs were identified in the leaves of Chinese kale (Table 6). By comparing our findings to those of Sun et al. (2011) and Qian et al. (2016) on Chinese kale sprouts (6-days-old), we identified four indole types [glucobrassicin (GBS), 4-OH glucobrassicin (4HGB), 4-methoxy glucobrasscin (4MGB), neoglucobrasscin (NGBS)] that were consistent with those found in our study, and two aliphatic types [SIN, gluconapin (GNP)] that were almost identical.
Table 6.
The major glucosinolates (GSLs) identified and the molecular weight (MW) of each GSL in the leaves of Chinese kale by mass spectrometryThe percentage of each GSL relative to total GSLs was 56 ‑ 79%, 8 ‑ 19%, 2 ‑ 10%, 2 ‑ 6%, 2 ‑ 5%, and 1 ‑ 11% for SIN, GBS, GNP, 4HGB, 4MGB, and NGBS, respectively. SIN content accounted for the highest percentage of the total GSLs, and was followed by GBS (Fig. 2). Previously, the content of SIN in Chinese kale sprouts (cv. DFZC; 6-days-old) was reported to be 15 ‑ 22% of the total GSLs (Qian et al., 2016); however, in the present study, that of Chinese kale (42-days-old) was found to be 56 ‑ 79%. Lee et al. (2016) reported that total GSL content of kale (B. oleracea var. acephala L.) harvested approximately 70 days after sowing was highest with B1:R2:W3, and among GSLs, GBS was the highest, followed by glucoiberin (GLB) and SIN. The content of SIN in kale (70-days-old) was reported to be approximately 16% of the total GSL. However, in a previous study (Qian et al., 2016), the absolute content of SIN in Chinese kale sprouts (6-days-old) was estimated to be 100-fold higher than that of the SIN of Chinese kale (42-days-old) in the present study (Table 7). Moreover, the absolute content of SIN in Chinese kale was approximately 68-fold higher in this study than that found in 70-days-old kale in another research (Lee et al., 2016). Thus, the content of GSLs may differ according to the growth stage and kale variety. In addition, it was estimated to decrease as growth proceeded.
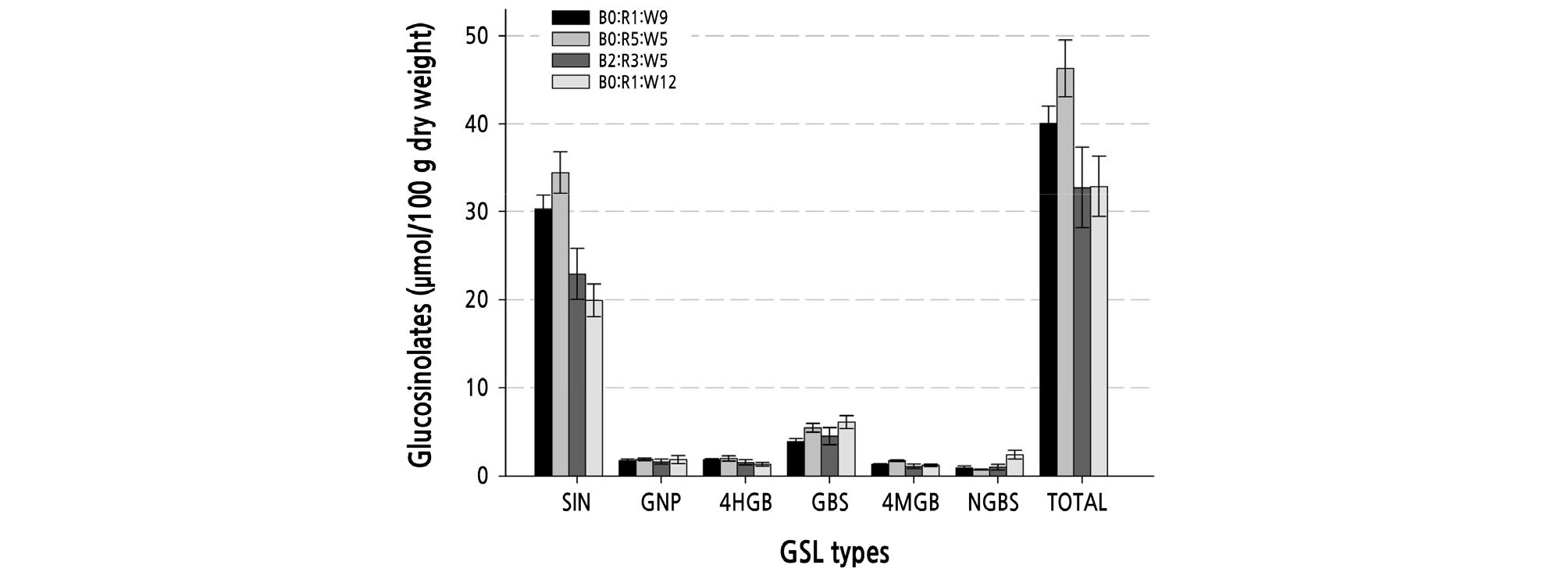
Fig. 2.
The contents of seven types of glucosinolates and the total glucosinolates in Brassica oleracea var. alboglabra Bailey cv. Jellujon. B0:R1:W9 (128 µmol·m-2·s-1, 20W); B2:R3:W5 (119 µmol·m-2·s-1, 20W); B0:R5:W5 (128 µmol·m-2·s-1, 20W); B0:R1:W12 (136 µmol·m-2·s-1, 24W). Significant differences at p = 0.05 by Duncan’s multiple range test. Values shown refer to those from Table 6.
Table 7.
Variety contents of glucosinolate and the total glucosinolate in Brassica oleracea var. alboglabra Bailey cv. Jellujon influenced by the combination of (NH4)2SO4 and LED at the harvest stagezyMean separation within columns by Duncan's multiple range test at p = 0.05.
xAbsolute and percentage of each glucosinolate content in total GSL.
ns,*,**,***Non-significance or significance at p ≤ 0.05, 0.01, or 0.001, respectively.
By comparing LED treatment alone, we found that B0:R5:W5 caused the highest total GSL content, followed by B0:R1:W9, B0:R1:W12, and B2:R3:W5 (Table 7). However, a significant difference was not found in the total GSL according to the (NH4)2SO4 concentration. Furthermore, total GSL contents were found to be significantly different among the 16 combinations described above, but no significant differences were found in the contents of six GSLs according to the (NH4)2SO4 concentration. The contents of SIN, 4MGB, and NGBS, with the exceptions of GBS, GNP, and 4HGB, were significantly different among the LED conditions. However, in the 16 combinations, very significant differences were identified for four GSLs, except GNP and 4HGB. SIN content was the highest at 40.9 µmol·100g-1 DW using the combination of S4 and B0:R5:W5, followed by the S2 and B0:R5:W5 combination (Table 7). GBS, the second highest content in Chinese kale, achieved the highest value of 7.9 µmol·100g-1DW with the combination of S2 and B0:R1:W12, followed by S3 and B2:R3:W5. Thus, the optimal combination for SIN content differed from that required for GBS content.
Deng et al. (2017) reported that treatment with red light at 1 ‑ 3 days prior to the harvesting of Chinese kale (cv. DSCH) could effectively maintain the GSLs known as anti-cancer substances such as GNP, GLB, and progoitrin. In the kale shoot tissues, SIN responded to wavelength treatment. In particular, the 640-nm and 730-nm wavelength treatments resulted in high SIN contents, but other wavelength treatments did not affect its accumulation (Lefsrud et al., 2008). Based on the high GSL content of Chinese kale in this experiment, we estimated that a combination treatment (B0:R5:W5), which had the highest proportion of red in LEDs, maintained or delayed the decrease in GSL content during the growth stage.
The isolation and identification of total GSLs can vary depending on plant varieties containing GSL and light quality. Ezo-wasabi showed high GNP and glucoibarin under single red LED and high GBS under blue LED among the total isolated GSLs (Abe et al., 2015). In addition, rocket salad (Eruca sativa L.) produces dimeric 4-mercaptobutyl, which causes strong and pungent aromas, was highly detected under white LED treatment (Lee et al., 2017). In this study, the content of SIN among the aliphatic types accounted for most of the total GSLs under red LED light. These results may be applied to efficiently accumulate GSL by red and blue LEDs depending on the crop. However, since the red LED is about 5 times more expensive than the white LED, it is suggested that when combining the red LED and the white LED, the number of white LED is increased to secure an appropriate light intensity, thereby economically promoting the growth and GSL content of Chinese kale.
Because all GSLs contain at least two sulfur groups, the supply of sulfur has a strong influence on GSL concentration. Jóska et al. (2009) reported that without supplementary sulfur, the GSL content in Indian mustard seed might decrease. In addition, it was assumed that the sulfur status was insufficient for genotype-specific GSL formation. In the absence of supplemental sulfur, different nitrogen supplies did not affect the concentration of SIN because of the absolute deficiency in sulfur. Furthermore, increasing nitrogen supply at the highest sulfur supply did not reduce the GSL concentration of white mustard (Sinapisabla) seeds (Josefsson, 1968). Therefore (NH4)2SO4 was found to have a greater effect on SIN based on the nitrogen and sulfur content present in plant GSLs. Enhancing the supply of sulfur is expected to reduce the degradation of GSLs. Although we did not identify a statistical difference in all GSL concentrations with (NH4)2SO4 treatment alone, except for 4HGBS, the decrease in GSL concentrations due to the aging of Chinese kale was postulated to delay the decrease in SIN relative to other GSLs because of the steady input of sulfur. In conclusion, the combination of S4 and B0:R5:W5 is deemed the most suitable for increasing total GSLs and the main functional substance of Chinese kale, SIN.