Introduction
Materials and Methods
Seed and Fungal Strains
DNA Extraction, Sequencing, and Phylogenetic Analysis
Morphology and Growth of Colonies
Experimental Design
Plant Growth Responses and Statistical Analysis
Results
Molecular Phylogenetic Analysis
Morphology and Growth Rate of the Three Isolates
Morphology and Colonization of Resynthesized Seedlings of R. kanehirae
Growth Responses of R. kanehirae
Discussion
Introduction
The genus Rhododendron belongs to the family Ericaceae (heath family), with as many as 1024 species, and consists of 850 ‑ 1,000 subspecies (Li et al., 1998; Fayaz, 2012). There are 23 native Rhododendron species in Taiwan, of these, 16 (70%) are endemic (Gibbs et al., 2011; Argent, 2015; MacKay and Gardiner, 2017). One of the endemic species, R. kanehirae, is now only present in the wild, and a small population is distributed along Beishih Brook in north Taiwan (Tseng, 1993). It is also listed as extinct in the wild by the Red List of Vascular Plants of Taiwan (Editorial Committee of the Red List of Taiwan Plants, 2017).
A mycorrhiza is an association between fungus and plant roots, a symbiotic relationship common in nature (Frank, 1885; Kirk et al., 2001). The various forms of mycorrhizal associations have roles in plant nutrition and nutrient cycling and influence the structure and dynamics of plant communities (Cairney and Meharg, 2003). For example, ericoid mycorrhizae (ERM) exhibit associations that are saprophytic (Rice and Currah, 2001; Piercey et al., 2002; Lin et al., 2011), mutualistic (Schmid et al., 1995), and tolerant (Bradley et al., 1981, 1982; Gibson and Mitchell, 2005; Lin et al., 2011). Ericoid mycorrhizal fungi (ERMF) positively influence growth, survival, and competitiveness by enhancing nutrient uptake for the host species (Vohník et al., 2005; Lin et al., 2011, 2019), and ERM can improve the host plant’s competitiveness at the ecosystem level (Michelsen et al., 1996).
Both organic and inorganic nitrogen can aid the regeneration and development of plants (Miller and Cramer, 2005), whereas inorganic nitrogen (such as ammonium and nitrate) can be directly absorbed by plants. A majority of plants absorb nitrogen from the soil as nitrate (NO3-) and ammonium (NH4+) (Marschner, 2012), but ericaceous plants prefer ammonium (Haynes, 1986). ERMF has demonstrated improved nitrogen uptake in its host plants (Read, 1996; Xiao and Berch, 1999; Cairney et al., 2000; Wei et al., 2016) and increases the seed germination rate of R. kanehirae to 60% (Lin and Wang, 2017).
It has also been reported that R.kanehirae seedlings can form an ERM with the fungus Oidiodendron maius (Lin et al., 2017), and while ericaceous plants are known to prefer to utilize ammonium, it is unclear what part the ERMF plays. The purpose of this study is to examine the ability of R.kanehirae seedlings to utilize inorganic nitrogen in the presence of three O. maius isolates. We also show that the isolate RhYM3 is a member of O. maius, and its combination with nitrogen effectively promotes the growth of R. kanehirae and achieves the effect of conservation.
Materials and Methods
Seed and Fungal Strains
Seeds of R. kanehirae were gathered in the Wu-Shi-Keng area in central Taiwan (120°56'53.5"E 24°16'24.5"N; altitude, 1,008 m). The following three isolates of hyphomycete O. maius were used in this study: CBS110450, RhYM3 (MU30559), and RooDK1 (MU30557). These strains were deposited at the Bioresource Collection and Research Center (BCRC), Hsinchu, and the Tree Mycorrhiza Laboratory of National Chiayi University, Taiwan. Strains CBS110450, RooDK1, and RhYM3 were isolated from a species of Vaccinium, R. ovatum, and R. hyperythrum, respectively, and belonged to O. maius (Zijlstra et al., 2005; Wang and Lin, 2014; Chien, 2016).
DNA Extraction, Sequencing, and Phylogenetic Analysis
Total DNA of the three isolates was extracted using a CTAB method (Doyle and Doyle, 1990) from mycelium growing on the surface of potato dextrose agar (PDA). Internal Transcribed Spacer (ITS) regions were amplified by PCR with the universal rDNA primers V9G (Gerrits van den Ende and Hoog, 1999) and LR1 (Vilgalys and Hester, 1990). A 25-µL reaction mixture contained 1.0 µM of each primer, 1.0 unit of Taq DNA polymerase (Fermentas, USA), 100 µM of each of the four deoxynucleotides, in a PCR buffer, and 0.25 µg of fungal DNA. The DNA target was amplified for 35 cycles of DNA denaturation at 94°C (10 min for the first cycle and 30 s for subsequent cycles), 1 min at 55°C for primer annealing, and 1 min at 72°C for DNA extension. The efficiency of amplification was monitored by running a 5-µL sample of each reaction mixture on a 1.2% agarose gel (Amresco, USA) at 125 V in Tris-borate-EDTA buffer. A 100-bp molecular weight ladder was used as the size standard. Ethidium bromide was used to stain the gel, which was visualized and photographed under ultraviolet light. The rDNA ITS region was sequenced by Tri-I Biotech Inc., Taipei, Taiwan.
Related sequences were searched by the BLAST method. The outgroup Pseudogymnoascus roseus and sequence data for the ITS regions were analyzed together (GenBank accession no. AF062819). Moreover, various species of Oidiodendron were also included for the analysis of the ITS regions. The sequences of the ITS regions were aligned using Clustal X 1.83 (Thompson et al., 1997) and manually adjusted with the BioEdit Sequence Alignment Editor (Hall, 2011). Maximum likelihood analysis was performed with the default settings using the same aligned datasets as the ones based on the Tamura-Nei model and 1000 bootstrap replications in MEGA 7.0 (Hall, 2013).
Morphology and Growth of Colonies
The three O. maius isolates were transferred to 2% malt extract agar (2%MEA: 20 g·L-1 malt extract, 15 g·L-1 agar, BD Bioscience PharMingen, San Jose, CA), PDA (39 g·L-1, BD), and cornmeal malt yeast agar (CMMY: 25 g·L-1 cornmeal, 10 g·L-1 malt extract, 2 g·L-1 yeast extract, 15 g·L-1 agar, Difco) plates and incubated in growth chambers at 23°C. The colony growth rates were measured after 14 days, and the cultural characteristics of the colonies were observed daily (Stoyke and Currah, 1991; Hambleton and Currah, 1997; Usuki and Narisawa, 2005).
Experimental Design
This study explores the optimal combination of inorganic nitrogen and O. maius isolates used with R. kanehirae. The observations were from a factorial experiment with two factors; one factor was an inorganic nitrogen with two treatments (ammonium and nitrate) and the other factor was O. maius isolates with four treatments (Control, CBS110450, RhYM3, and RooDK1). Three replicated specimens that have a growing influence on R. kanehirae were constructed and tested for the eight treatment combinations. The 24 specimens were prepared and tested in random to adhere to a completely randomized design.
These strains were semi-pure and resynthesized according to the Dalpé method (1986). After surface cleaning, R. kanehirae seeds were sterilized with 35% hydrogen peroxide solution (H2O2) for 1 min and rinsed three times with sterilized distilled water. Then, they were transferred to a container with peat for germination. Germinated seedlings were transplanted into a plug system with a nursery substrate (peat and perlite 1:1 v/v, previously autoclave-sterilized at 121°C for 60 min). After cultivation for seven days, the seedlings were inoculated with/without O. maius isolates (four treatments) and grown in a growth chamber (22°C and 16 h of light at a maximum illumination of 5,000 lux). Standard fertilizer was modified from Mitchell and Read (MMR) (Dalpé, 1986) nutrient solution, 5 ml of standard fertilizer was watered on Monday and Wednesday, and watered with distilled water every day. Two formulations of MMR nutrient solution were prepared to measure how inorganic nitrogen affected growth. Ammonium is a standard MMR nutrient solution (NH4Cl, 32 mg·L-1; CaCl2·7H2O, 43.5 mg·L-1; MgSO4·7H2O, 10 mg·L-1; KCl, 5.5 mg·L-1; FeCl3, 3.75 mg·L-1; sucrose, 2 g·L-1; KH2PO4, 210 mg·L-1; pyridoxine, 100 µg·L-1; and thiamine, 100 µg·L-1). Nitrate is a modified MMR nutrient solution except with the NH4Cl in the ammonium replaced by KNO3 (Cairney et al., 2000). Two months after incubation, seedlings were removed, observed, and analyzed by the methods described below.
For all treatments, roots were collected and cleaned with water in an ultrasonic oscillator. For staining, roots were cleared in 10% KOH for 24 h, washed in dH2O, acidified in 10% HCl for 1 h, transferred into 0.05% aniline blue (aniline blue, 0.25 g; dH2O, 25 mL; lactic acid, 475 mL) for 1 h, and then destained in the destaining solution (dH2O, 25 mL; lactic acid, 475 mL) for 2 h. Roots were then mounted on microscope slides in lactoglycerol (14:1:1, lactic acid:glycerol:dH2O) for observation (Upson et al., 2007).
Plant Growth Responses and Statistical Analysis
To measure the effects of all treatments on the growth performance of seedlings, the seedling growth media were carefully removed after 2 months of incubation. The heights (measured from the cotyledon scar to the base or tip of the terminal bud) and weights of fresh roots and shoots were measured. The shoot-root (SR) ratio (Harris, 1992) is a simple tool to evaluate seedling quality that consists of several parameters: weights of fresh roots (RFW, mg) and shoots (SFW, mg). SR ratio is calculated as follows:
SR ratio = SFW (mg) / RFW (mg)
Data were statistically analyzed using Levene’s test to check the homogeneity of variances. Next, a normal probability plot of residuals and the Shapiro-Wilk test were used to check the normality assumption. A two-way ANOVA was used to evaluate the effect of the inoculation on the heights and weights of the fresh roots and shoots. This experiment has two control factors: (i) inorganic nitrogen (ammonium and nitrate) and (ii) O. maius isolates (Control, CBS110450, RhYM3, and RooDK1). Significant differences between treatments groups were evaluated by multiple pairwise comparison using Tukey’s HSD with p ≤ 0.05. R software was used for statistical analysis.
Results
Molecular Phylogenetic Analysis
To verify the species of the three isolates used in this study, we obtained the sequences of the ITS II region of the isolates and other Oidiodendron species and constructed a maximum likelihood phylogenetic tree. As shown in the phylogenetic tree (Fig. 1), isolates CBS110450, RhYM3, and RooDK1 were clustered from O. maius with strong bootstrap values (99%) and were significantly separated from sequences of other Oidiodendron species. Sequence data of RhYM3 from this study were deposited in GenBank under accession number MG890316 (Table 1).
Table 1.
Data on the three O. maius isolates used in this study, all of which were isolated from roots of an ericaceous host
Strain | Host plant | GenBank accession no. | Reference |
CBS110450 | Vaccinium vitis-idaea | FN812713 | Zijlstra et al., 2005 |
RhYM3 | Rhododendron hyperythrum | MG890316 | Chien, 2016 |
This study | |||
RooDK1 | Rhododendron ovatum | MF611694 | Wang and Lin, 2014 |
Lin et al., 2019 |
Morphology and Growth Rate of the Three Isolates
The three isolates were incubated on different media (2%MEA, CMMY, and PDA) for the morphological and growth rate studies (Fig. 2). After 14 days of incubation, the colonies of strain CBS110450 showed a white color (Fig. 2A ‑ 2C), with average colony growth rates of 1.10 ± 0.01, 1.15 ± 0.01, and 1.03 ± 0.03 mm d-1, respectively, for each culture medium. The colonies of strain RhYM3 cultured on the same media showed a white color (Fig. 2D ‑ 2F), with average colony growth rates of 0.76 ± 0.01, 0.74 ± 0.01, and 0.84 ± 0.02 mm d-1, respectively, for each culture medium. The colonies of strain RooDK1 cultured on the same media showed a white color (Fig. 2G ‑ 2I), with average colony growth rates of 1.24 ± 0.02, 1.10 ± 0.01, and 1.04 ± 0.01 mm d-1, respectively, for each culture medium. The colonies of these three isolates not only have statistically significant growth rates, but they also have different morphology types in the three types of media.
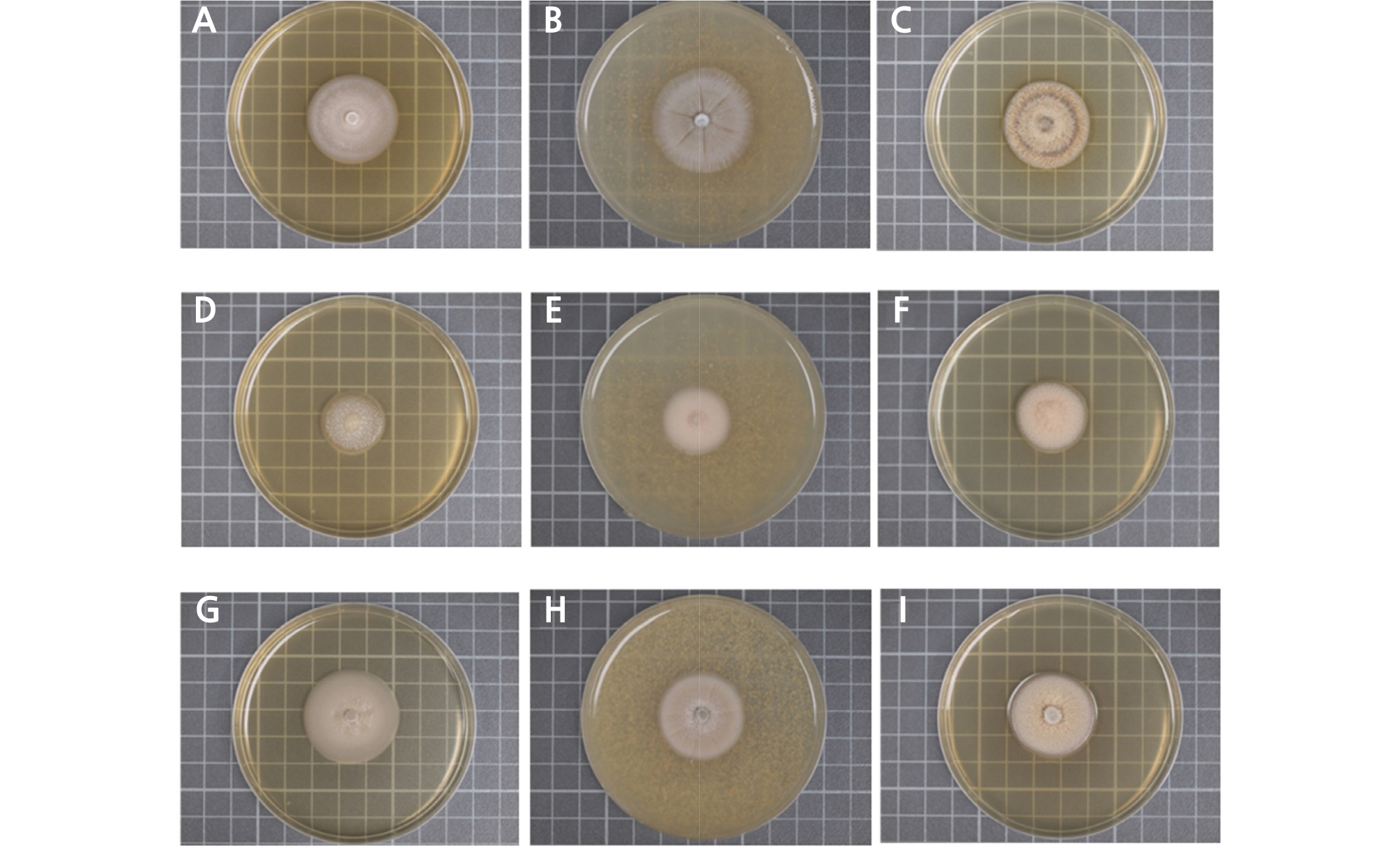
Fig. 2.
The morphology of three isolate colonies cultured on different media after 14 days. A–C: CBS110450 was cultured on 2%MEA, CMMY, and PDA media, respectively. D–F: RhYM3 was cultured under the same conditions. G–I: RooDK1 was also cultured under the same conditions. Each lattice unit is 1 cm2 (Continued).
Morphology and Colonization of Resynthesized Seedlings of R. kanehirae
For experimental methods and materials, see the section on morphology and growth of colonies. All seedlings survived after 2 months of incubation in the study (Fig. 3). The characteristics of root ‑ fungus associations for all treatments were observed by light microscopy and recorded (Fig. 4). The RhYM3-inoculated seedlings grew well (Fig. 3A and 3B), and hyphal complexes were visible in the stained roots (Fig. 4A and 4B); similar outcomes were observed for RooDK1 (Fig. 3C and 3D, Fig. 4C and 4D) and CBS110450 (Fig. 3E and 3F, Fig. 4E and 4F). In the controls, the seedlings also grew well (Fig. 3G and 3H), but no hyphae were found in the stained roots (Fig. 4G and 4H).
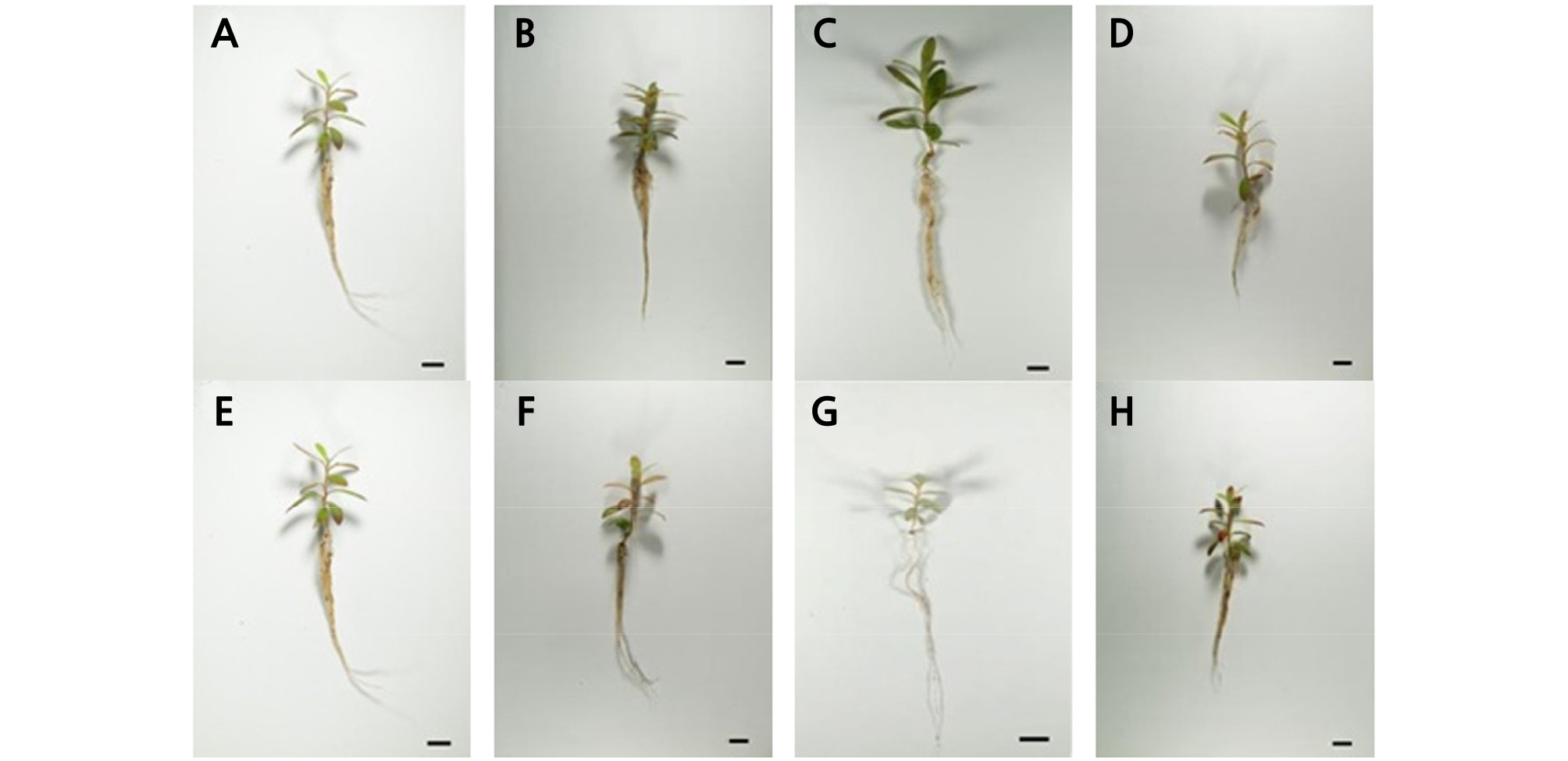
Fig. 3.
Inoculation of R. kanehirae with different fungal isolates and inorganic nitrate sources. A: RhYM3 inoculation with nitrate. B: RhYM3 inoculation with ammonium. C: RooDK1 inoculation with nitrate. D: RooDK1 inoculation with ammonium. E: CBS110450 inoculation with nitrate. F: CBS110450 inoculation with ammonium. G: Control without inoculation using nitrate. H: Control without inoculation using ammonium. Scale bar = 1 cm.
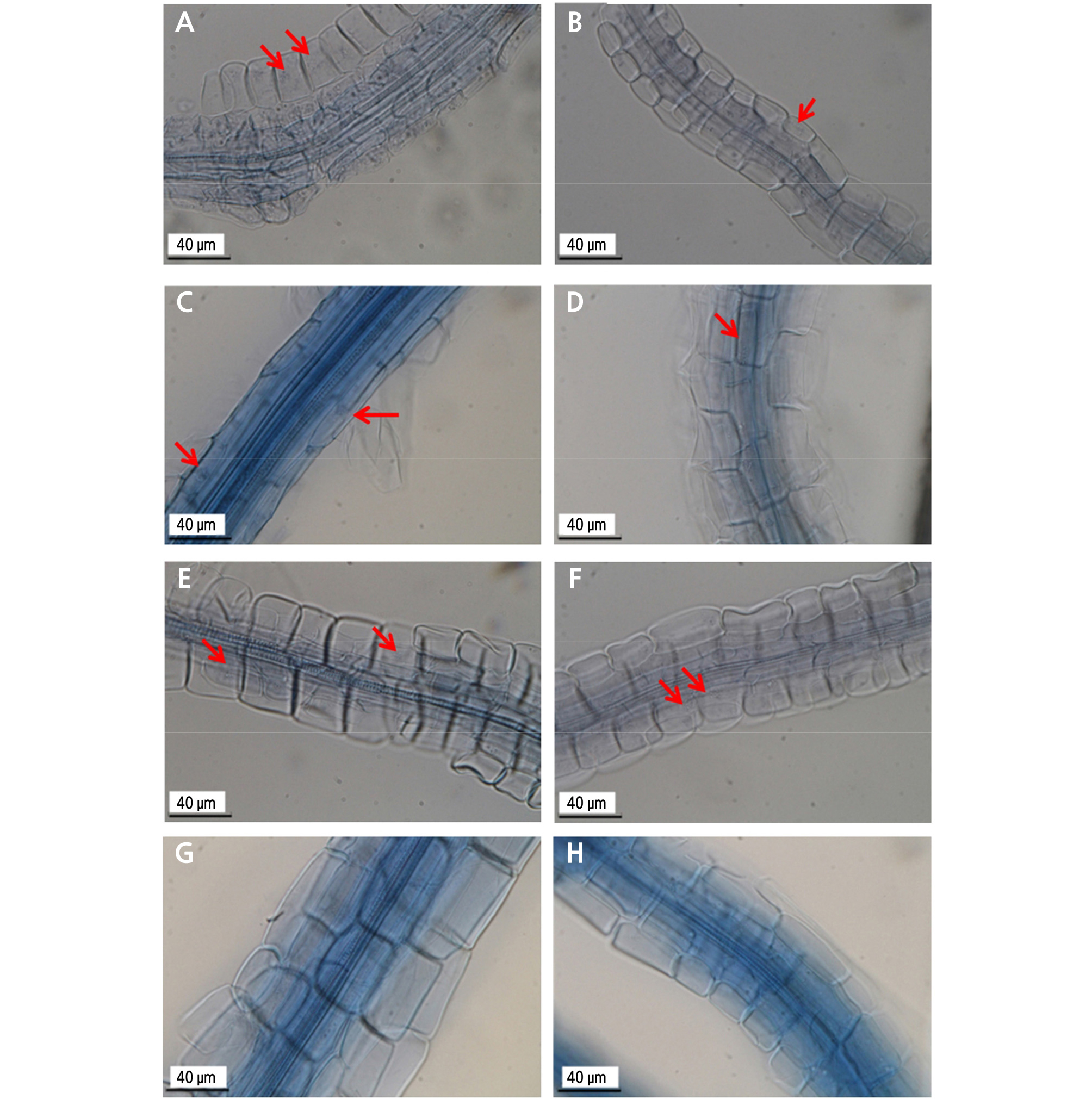
Fig. 4.
Roots of R. kanehirae seedlings grown either with nitrate or ammonium and stained to detect mycorrhizal strains (arrows showed hyphal complexes and with scale bar = 40 µm). A/ B is RhYM3 with nitrate/ammonium, C/D is RooDK1 with nitrate/ammonium, E/F is CBS110450 with nitrate/ammonium, and G/H is control with no fungal inoculation. Arrows point to hyphal complexes.
Growth Responses of R. kanehirae
Seedlings inoculated with each of the three fungal strains all showed improved growth after 2 months of incubation (Tables 2 ‑ 4 and Fig. 5). Two-way ANOVA was considered in this study; factor A was the treatment “inorganic nitrogen type” with levels “ammonium” and “nitrate,” and factor B was with three O. maius isolates (CBS110450, RhYM3, and RooDK1) and control (CK). The heights, fresh weights of shoots (SFW), fresh weights of roots (RFW), and total fresh weights (TFW) showed significant differences with different inorganic nitrogen (factor A). For factor B, significant differences were observed for fungal strain effects in SFW, RFW, and TFW, but not in height. Significant interaction effects were observed for RFW and TFW, but not height and SFW. The interaction effects were probably less important, since they were small compared to the two main effects (Table 2).
Table 2.
The significance of the differences in plant parameters resulting from the interactions among factors A (ammonium and nitrate) and B (fungal strains CBS110450, RhYM3, RooDKl, and CK)
Source | A | B | A×B |
Height | 41.380 *** | 2.487 NS | 2.527 NS |
Root FW | 33.751 *** | 19.795 *** | 4.259 * |
Shoot FW | 15.258 ** | 7.052 ** | 3.007 NS |
Total FW | 46.523 *** | 23.403 *** | 5.851** |
Table 3.
Height and fresh weight of R. kanehirae seedlings with different treatments after two months of incubation
Table 4.
The shoot-root (SR) ratio of R. kanehirai seedlings with different treatments after two months of incubation
Treatment | SR ratio | |
Nitrate | Ammonium | |
CBS110450 | 2.53 ± 0.07z by | 1.50 ± 0.20 a |
RhYM3 | 1.67 ± 0.21 b | 1.97 ± 0.47 a |
RooDK1 | 16.03 ± 4.01 aA | 2.57 ± 1.32 aB |
Control | 7.10 ± 3.20 b | 2.43 ± 1.10 a |
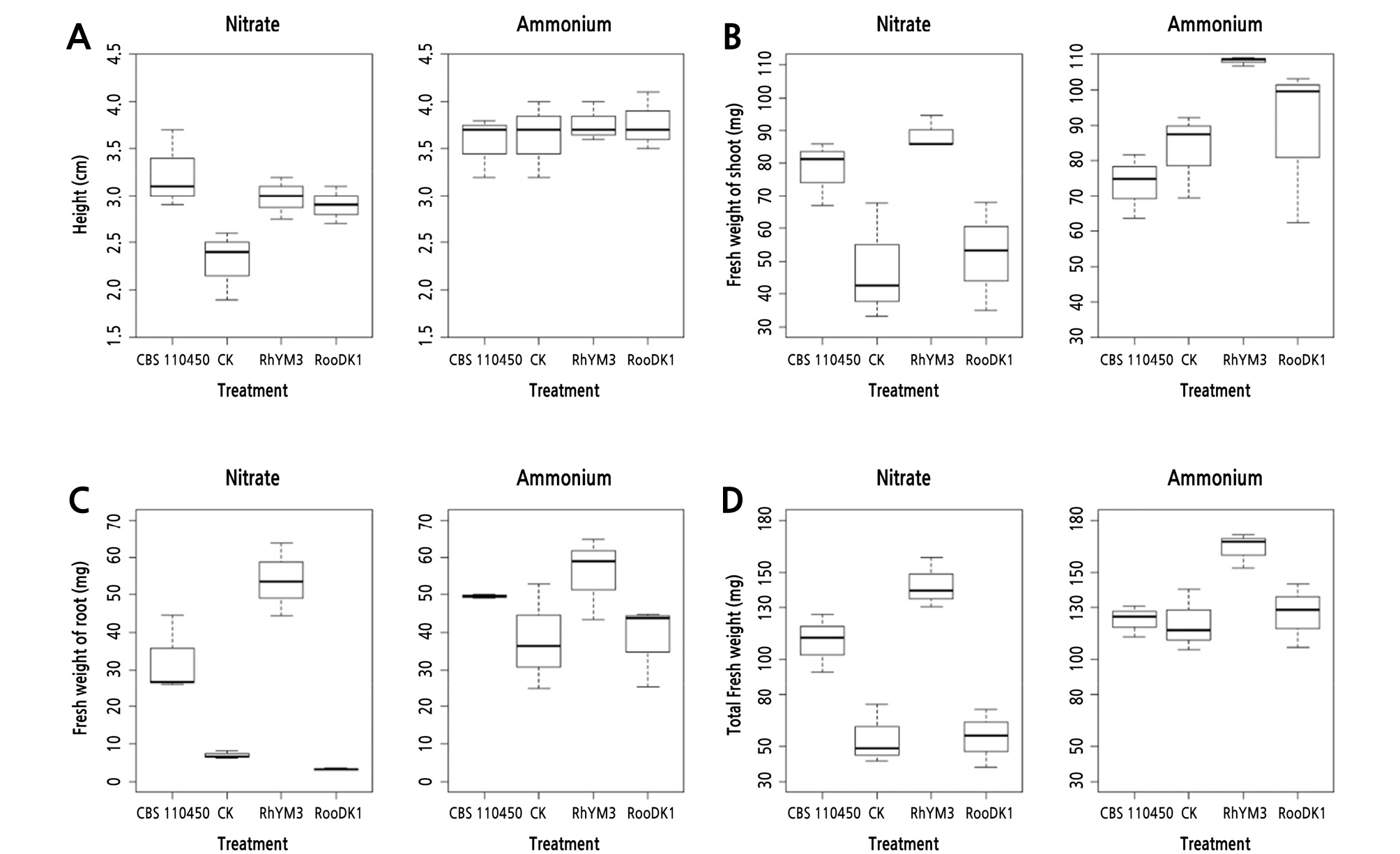
Fig. 5.
Boxplots showing R. kanehirae seedling height (A), fresh weight of shoot (B), fresh weight of root (C), and total fresh weight (D) as influenced by nitrogen supply (nitrate and ammonium) and Oidiodendron maius isolates (RooDK1, CBS110450, RhYM3, and CK) after incubation for 2 months. CK indicates control.
All inoculated combinations with nitrate treatment showed significant differences in seedling heights compared to the control, with measurements for CBS110450, RhYM3, RooDK1, and control of 3.23 ± 0.42, 2.98 ± 0.23, 2.90 ± 0.20, and 2.30 ± 0.36 cm, respectively. Conversely, all inoculated combinations with ammonium treatment showed no significant differences in seedling heights, with measurements for CBS110450, RhYM3, RooDK1, and control of 3.57 ± 0.32, 3.77 ± 0.21, 3.77 ± 0.31, and 3.63 ± 0.40 cm, respectively. Comparing the two inorganic nitrogen types for each fungal strain and control, ammonium improved seedling height significantly better than nitrate for RhYM3, RooDK1, and control treatments (Table 3).
Seedling shoot fresh weights (SFWs) of the nitrate treatment inoculated with different fungal strains showed that CBS110450 (88.77 ± 5.05 mg) and RhYM3 (78.00 ± 9.87 mg) had significant differences compared with RooDK1 (52.00 ± 16.58 mg) and control (47.80 ± 17.85 mg). Seedling SFW of ammonium treatment inoculated with different fungal strains showed that RhYM3 (108.07 ± 1.21 mg) and RooDK1 (88.27 ± 22.56 mg) had the same effect, but RhYM3 was significantly different compared to CBS110450 (73.33 ± 9.19 mg) and control (82.97 ± 12.06 mg). Comparing the two inorganic nitrogen types for each fungal strain and control showed that ammonium increased SFW significantly for both RhYM3 and control (Table 3).
Seedling root fresh weights (RFWs) of nitrate treatment combined with different inoculates and control showed that RhYM3 (54.00 ± 9.66 mg) had significant differences compared to CBS110450 (32.50 ± 10.48 mg), control (6.97 ± 1.08 mg), and RooDK1 (3.20 ± 0.26 mg), and control and RooDK1 were not significantly different (Table 4). Seedling RFW was no statistically significant different between different inoculates and control when combined with ammonium treatment. Comparing the two inorganic nitrogen types, ammonium increased seedling RFW more than nitrate significantly for RooDK1, CBS110450, and control treatments (Table 3).
The seedling total fresh weights (TFWs) of nitrate treatment combined with different inoculates and control were similar to RFW. RhYM3 had the highest value (142.73 ± 14.29 mg), followed by CBS110450 (110.50 ± 16.55 mg), RooDK1 (55.20 ± 16.82 mg), and control (54.77 ± 17.22 mg). TFW of ammonium treatment combined with different inoculates and control showed that RhYM3 (163.80 ± 10.00 mg) had significant differences compared to RooDK1 (126.33 ± 18.25 mg), CBS110450 (122.87 ± 8.96 mg), and control (121.10 ± 17.68 mg) (Table 3). Comparing the two inorganic nitrogen types, ammonium increased TFW more than nitrate, and there was no significant difference for CBS110450 and RhYM3.
The shoot-root ratio (SR) of R. kanehirai seedlings combined with RooDK1 and nitrate was 16.03, while the SR ratio of RooDK1 combined with ammonium was only 2.57 (the result is similar to control) (Table 4). These results indicated that R. kanehirae seedlings, with or without ERMF inoculation, exhibit increased growth in their root systems, especially in the presence of ammonium. Seedlings inoculated with strains CBS110450 and RhYM3 exhibit a better growth response overall (Table 3) and have a lower SR ratio (Table 4).
Discussion
Inorganic nitrogen can be absorbed directly by plants; nitrate is preferred by most plants (Marschner, 2012), and ammonium is preferred by ericaceous plants (Haynes, 1986). In this study (Table 4), TFW for R. kanehirae seedlings without ERMF inoculation (control) was 2.2 times higher with ammonium than with nitrate, and RFW was up to 5 times higher with ammonium than with nitrate.
ERMFs have been demonstrated to promote nitrogen uptake in host plants (Read, 1996; Wei et al., 2016). Among the three O. maius isolates used in this study, RooDK1 isolated from R. ovatum was demonstrated to be an O. maius strain (Wang and Lin, 2014) that improved growth of its host plant (Lin et al., 2019). R. kanehirae seedlings inoculated with strain RooDK1 and treated with nitrate showed the worst performance in terms of SFW, RFW, and TFW (Table 4), and the control group had the same result. Strain CBS110450 isolated from Vaccinium vitis-idaea was also identified as O. maius (Zijlstra et al., 2005). Our study showed that R. kanehirae seedlings treated with nitrate and CBS110450 had better SFWs, RFWs, and TFWs than the control group. However, there were no significant differences between nitrate and ammonium in terms of SFW and TFW, which indicates that strain CBS110450 enabled R. kanehirae seedlings to utilize nitrate, similar to arbuscular mycorrhiza (Bücking and Kafle, 2015). Strain RhYM3 was isolated from R. hyperythrum (Chien, 2016). RhYM3-inoculated R. kanehirae seedlings treated with nitrate had better SFWs, RFWs, and TFWs than the control (Table 3). TFW of R. kanehirae seedlings with RhYM3 treatment showed no significant difference between nitrate and ammonium, a result similar to CBS110450.
The root system is crucial for maintaining plant health because it allows plants to absorb water and nutrients from the surrounding soil. The shoot-root (SR) ratio is a measurement that assesses the overall health of a plant. The definition of SR ratio is the quotient of SFW divided by RFW of R. kanehirae. The SR ratio can be used to evaluate seedling quality. A lower SR ratio (Grossnickle, 2005a, 2012; Ivetić et al., 2016) and larger root system (Grossnickle, 2005b) are often associated with greater height and a higher survival rate of seedlings in the field.
The results of this study demonstrate that R. kanehirae seedlings prefer ammonium over nitrate as a mineral nitrogen source (Table 3) and that nitrate can inhibit root development of R. kanehirae seedlings in the absence of an ERMF (in Control and RhYM3 treatment of Table 4). Strains CBS110450 and RhYM3 help their host plant overcome the stress of nitrate and produce better growth, a result similar to those reported by Read (1996) and Wei et al. (2016). Ericoid mycorrhizal strains of O. maius isolated from different habitats have been shown to exhibit functional diversity (Martino et al., 2003). Among the three O. maius isolates investigated in this study, strain RhYM3 exhibited the best performance in terms of TFW and SR ratio.